[ 308,914 keer bekeken / views ]
Slimme Meter Uitlezer – Nieuwe Functionaliteit
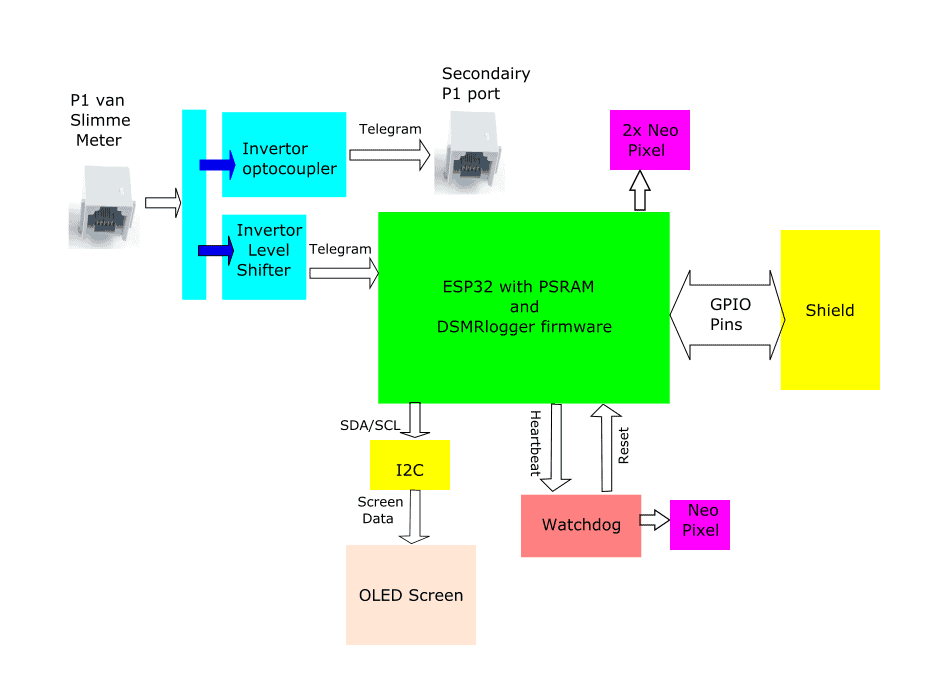
In deze post beschrijf ik nieuwe functionaliteit van de DSMRlogger32.
Continue Reading …
Overstappen naar PlatformIO (part 1)
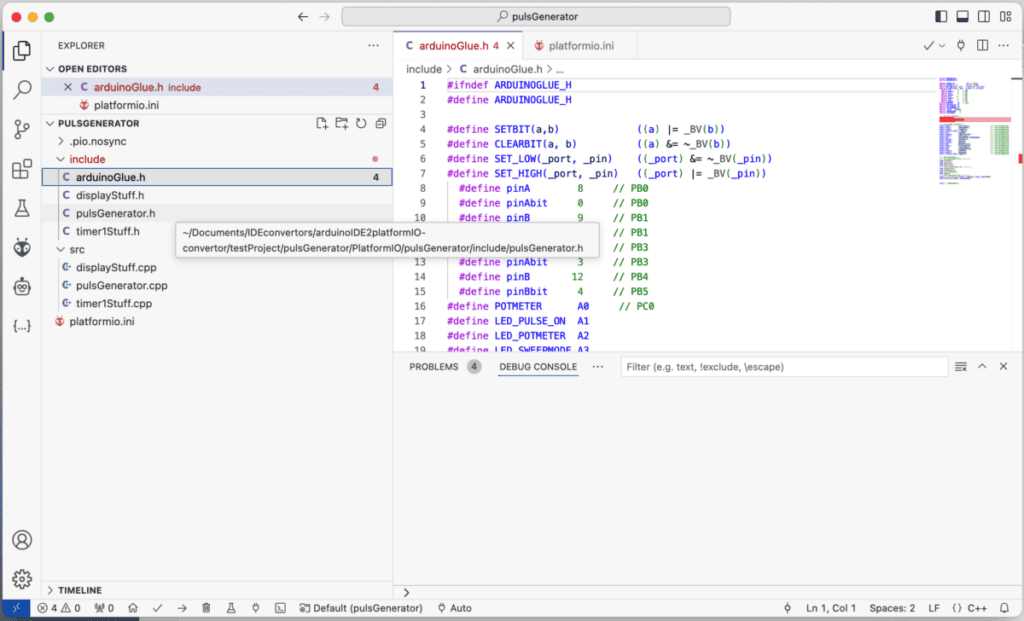
In deze post beschrijf ik een programma waarmee eenvoudig een Arduino IDE project omgezet kan worden naar PlatformIO
Continue Reading …
Overstappen naar PlatformIO (part 2)
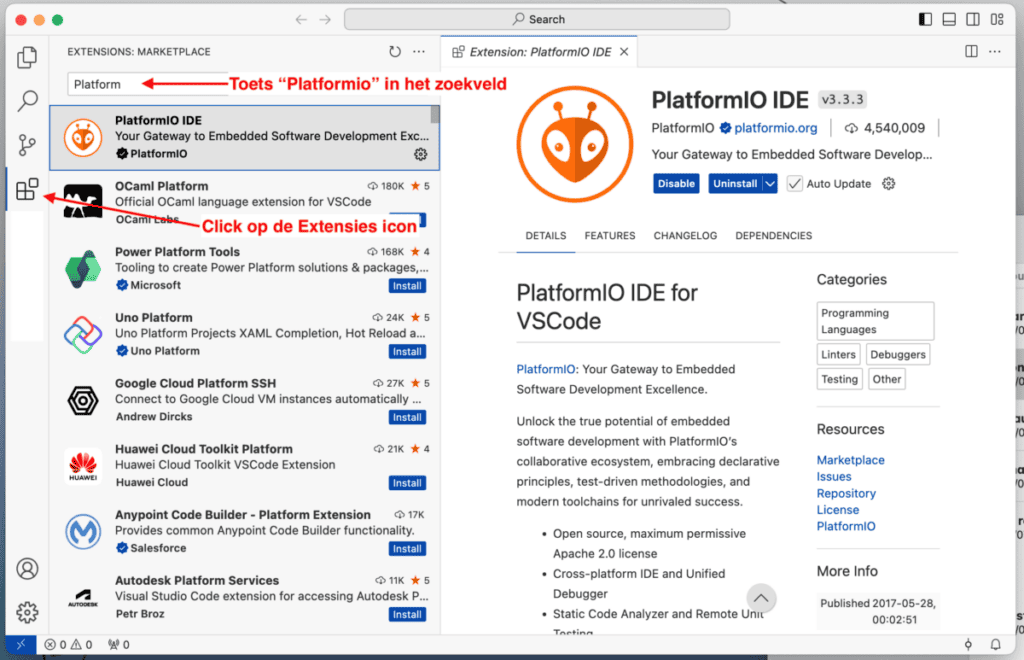
In deze post geef ik een korte introductie van de PlatformIO IDE
Continue Reading …
Universal Prototyping Board
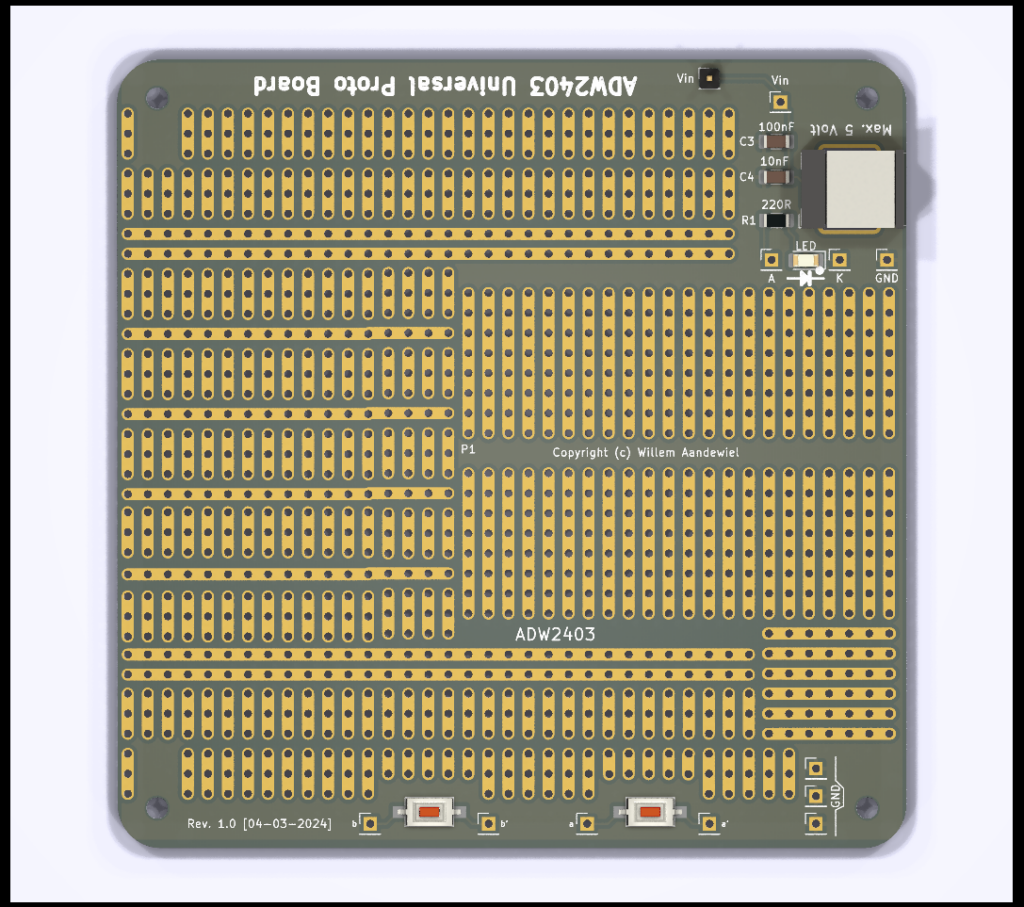
In the intricate world of microcontroller unit (MCU) development, versatility is often sacrificed for specificity. However, imagine a single solution that transcends these limitations – the Universal Prototyping Board.
Continue Reading ..
Virtuele P1 Kabel
Deze post beschrijft hoe je een “Virtuele P1 Kabel” kunt maken.
Continue Reading …
RGB LED strip controller
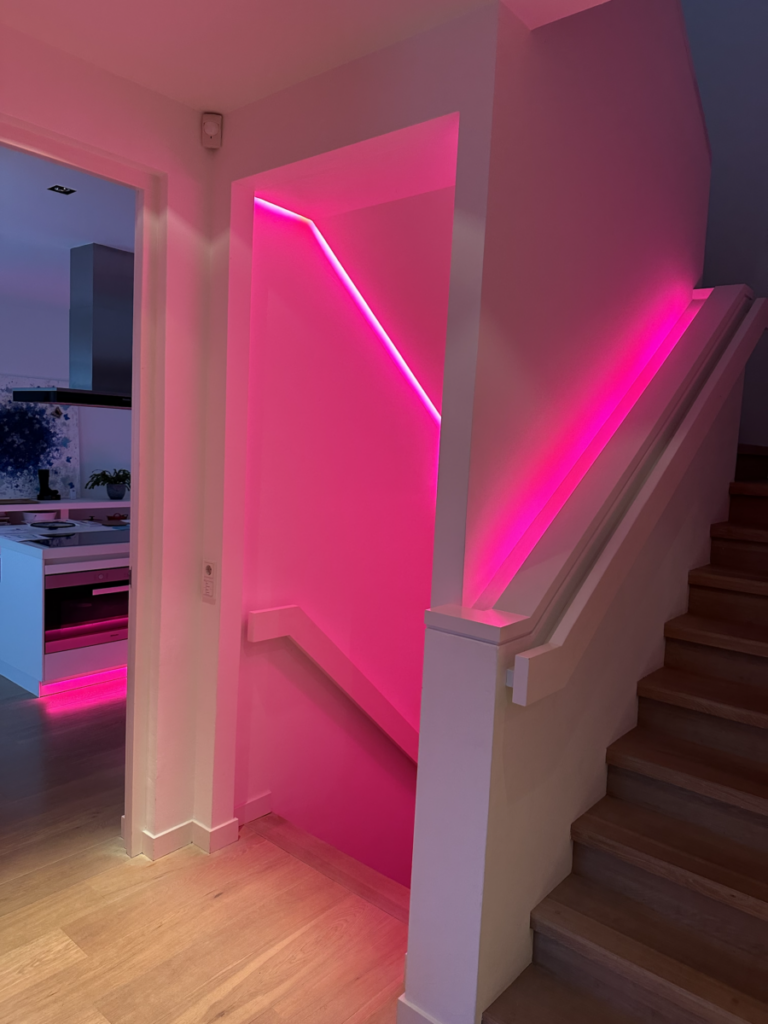
This project describes the design of a RGB LED strip controller board with an ESP32 processor board (ESP-DevKitC).
Continue Reading …
Crisis? What crisis? (nieuwe Slimme Meter reader)
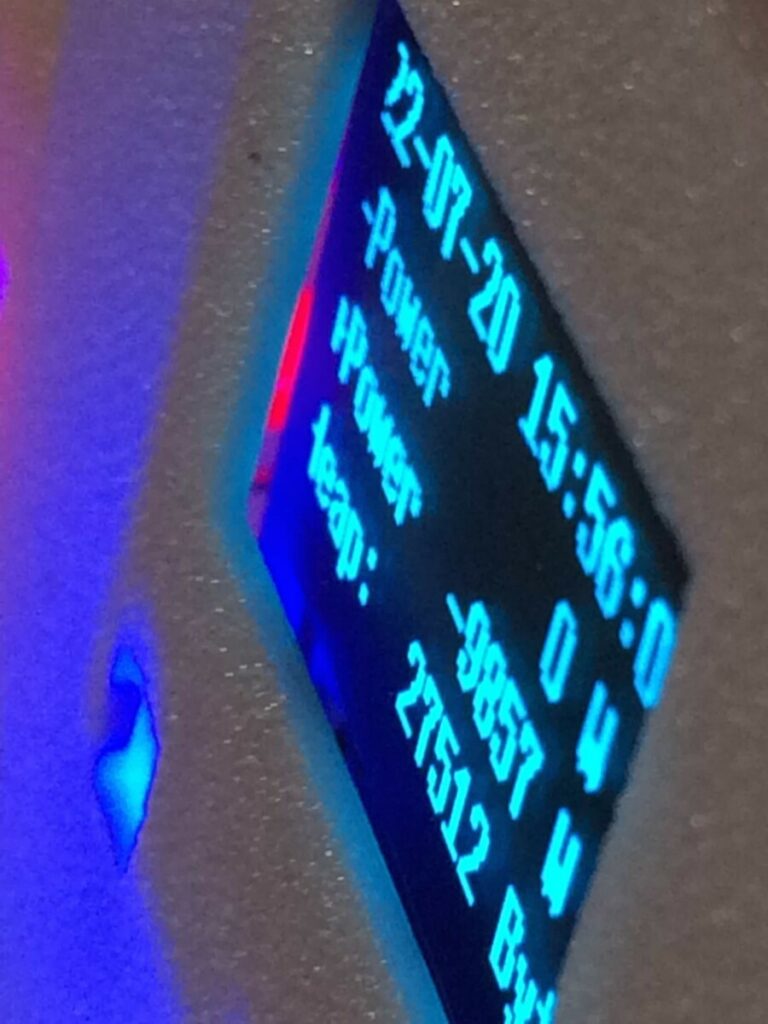
Met een energiecrises die iedereen raakt wordt het “in de gaten houden” van je energie verbruik steeds belangrijker. Daarom is het, na bijna vier jaar, hoog tijd om een nieuwe DSMR-logger te ontwikkelen.
Continue Reading …
Porting Tasmota naar de DSMR-logger32
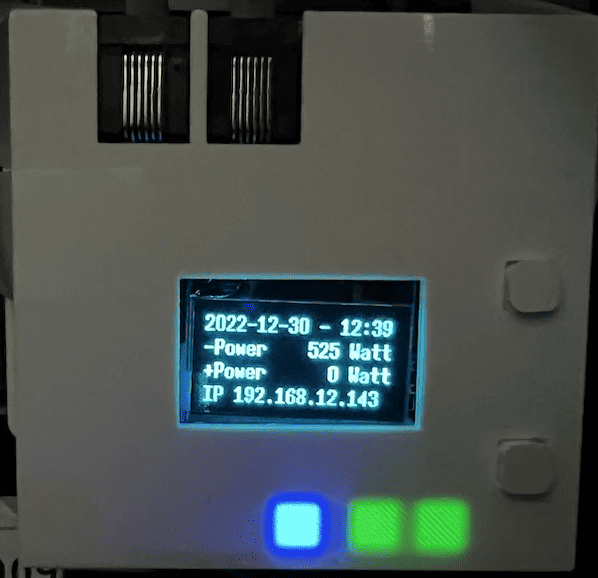
Omdat ik (Davy) thuis reeds heel wat esp8266 modules draaien heb met Tasmota, wenste ik de DSMR-logger32 ook te flashen met Tasmota.
Continue Reading …
Switch to PlatformIO (part 1)
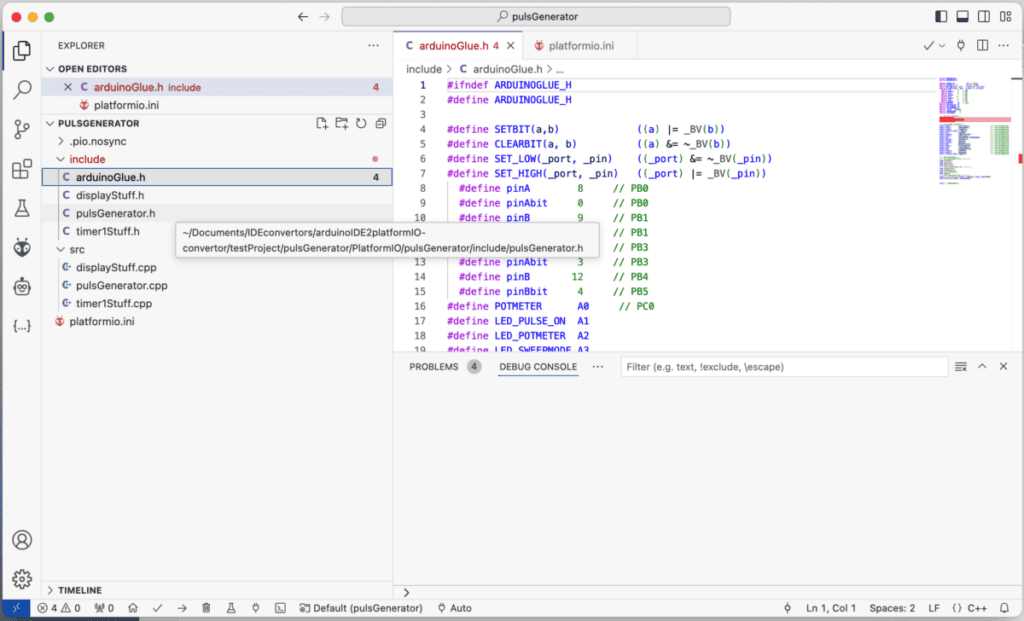
In this post I describe a program that can be used to convert an Arduino IDE project to PlatformIO
Continue Reading
MIDI Drum Machine
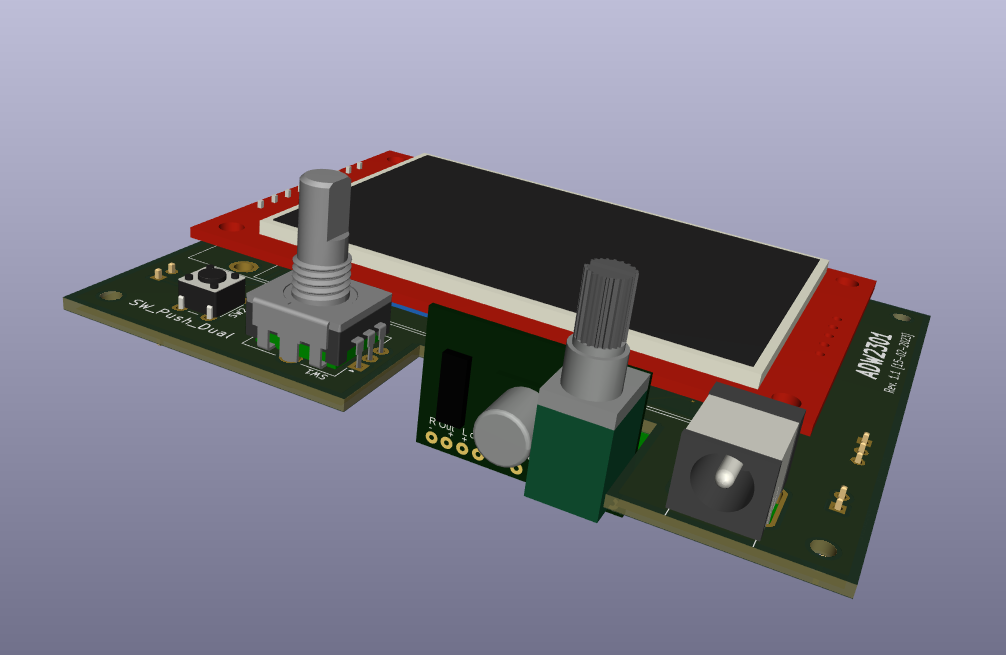
Een MIDI Drum Machine gebaseerd op een project van Peter Balch. Het is een één-op-één kopie van wat Peter Balch heeft gemaakt met alleen een door mij ontwikkelde PCB. Het is een leuke puzzel om alle onderdelen op een zo klein mogelijke printplaat te groeperen en dan ook nog zodanig dat je ze ook nog kunt solderen.
Continue Reading
Experimenteren met Micro Controllers
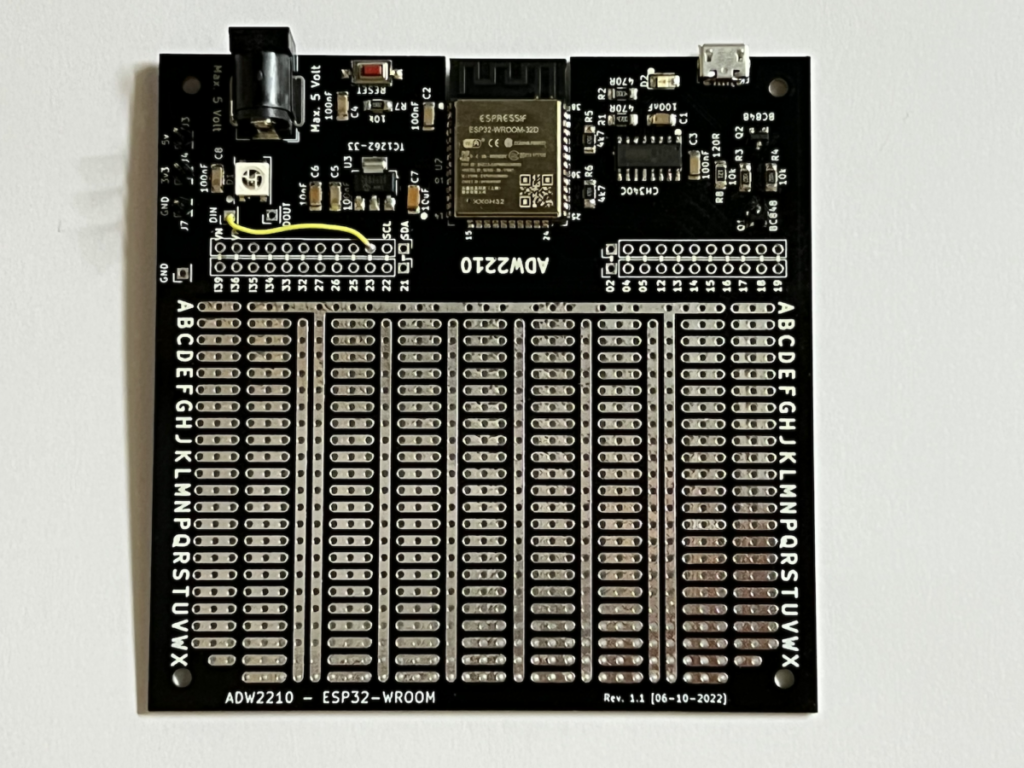
Ik heb twee nieuwe universeel toepasbare experimenteer printplaten ontworpen. Eén voor de ESP32 WROOM module en één voor de ESP32WROVER module. Op veler verzoek en voor het gebruiksgemak hebben beide bordjes een CH340C aan boord waarmee je de ESP32 eenvoudig via de USB poort kunt flashen. Als extra hebben ze ook een NeoPixel aan boord (gewoon, omdat het kán)..
Continue Reading ..
Zwerfafval detectie bij ondergrondse containers
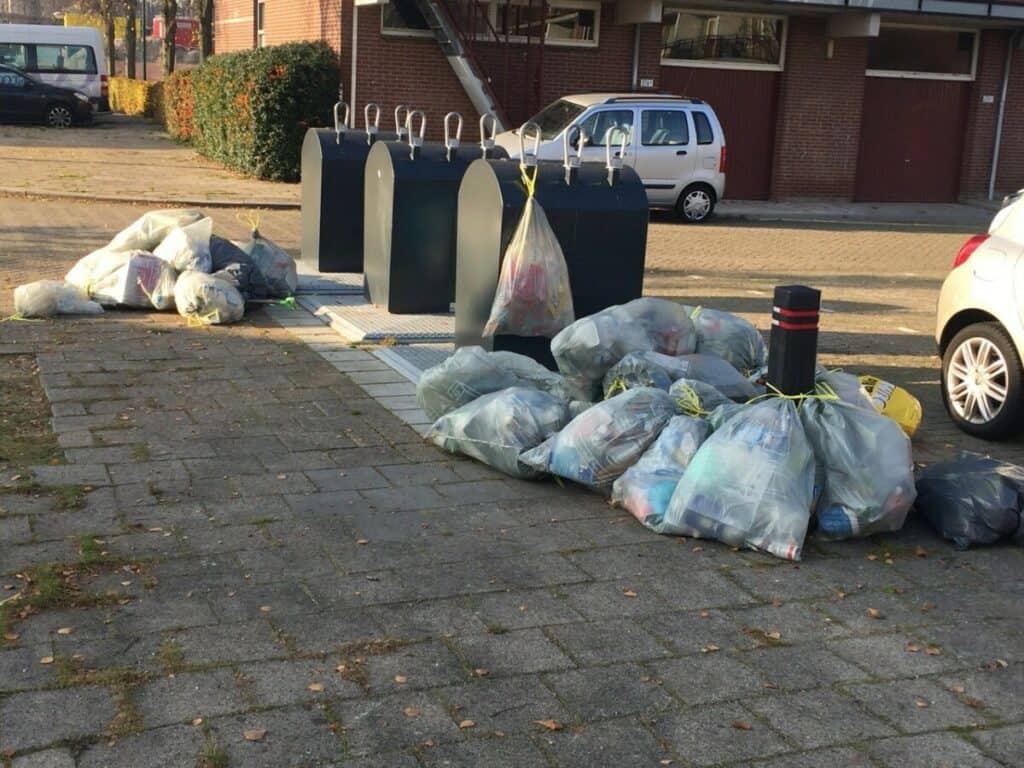
Deze post beschrijft de ontwikkeling van een Zwerfafval Detectie en Meldingen Systeem voor ondergrondse containers .. Continue Reading ..
Spotify Muziekspeler met Intuïtieve bediening
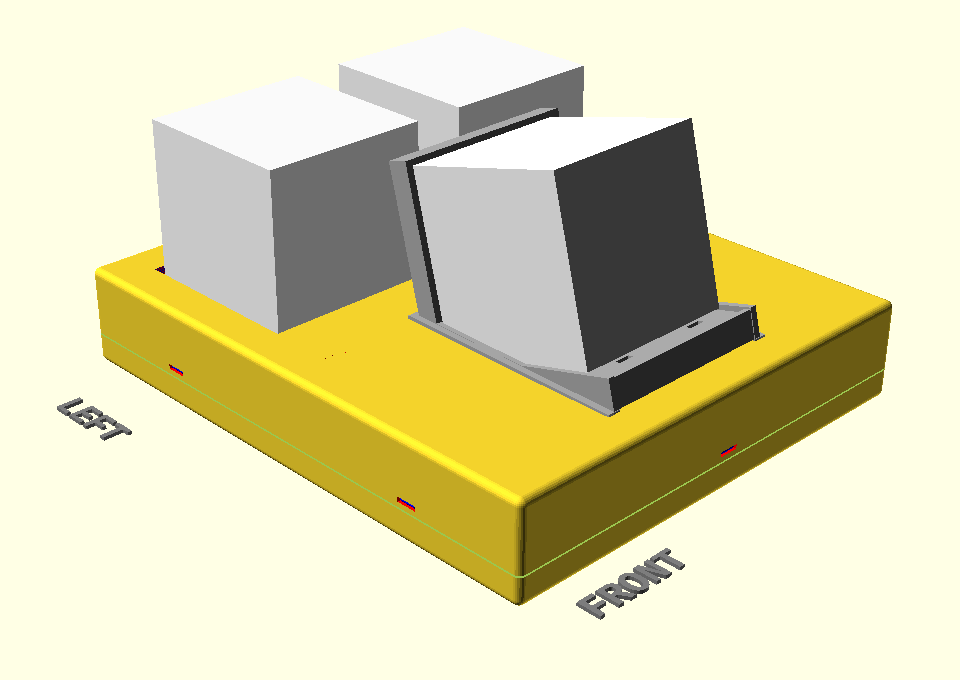
In deze post presenteer ik een project waarmee op een ándere manier muziek afgespeeld kan worden
Continue Reading ..
External Watchdog Timer
In this post I describe how to make an External Watchdog Timer to monitor, for instance, an ESP8266 or ESP32.
Continue Reading ..
Nog een geparametriseerde projectbox generator (YAPP)
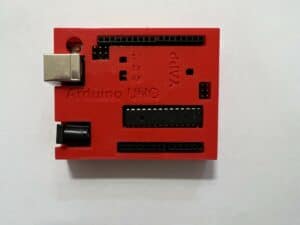
Yet Another Parametric Projectbox Generator
In deze post leg ik uit wat de uitgangspunten van deze YAPP generator zijn en hoe je hem kunt gebruiken om mooie projectboxen te 3D-printen!
Continue Reading ..
AT tester (Challenge/Response)
For a project I’m working on with Jelmer (JDJelectronics) we have to communicate with a SIMCOM GPRS modem.
Continue Reading ..
P1 Poort Extender (HUB)
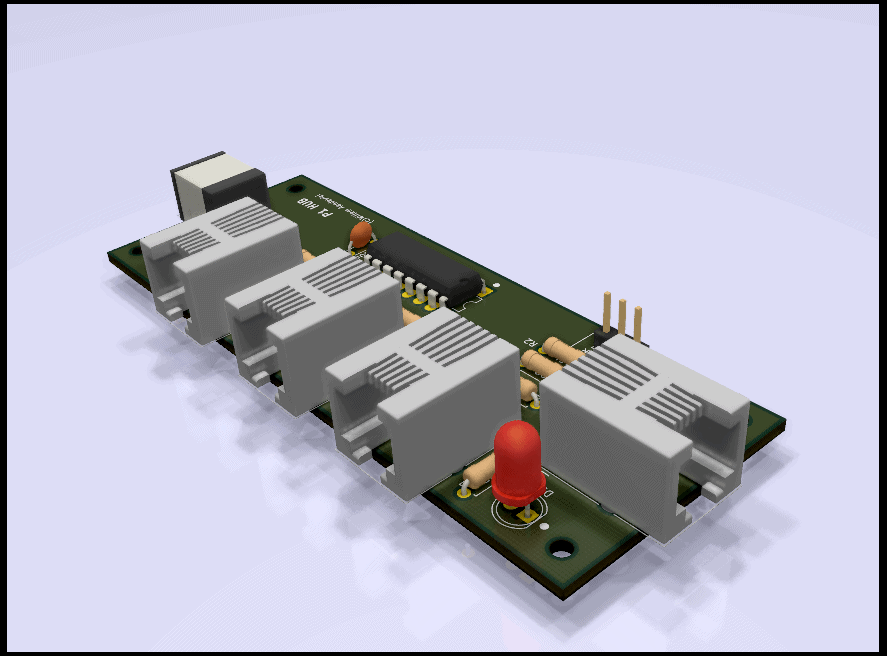
Omdat er steeds meer Slimme meter uitlees kastjes op de markt komen is één P1 poort niet altijd genoeg, maar door de opzet van de P1 poort is het niet zonder meer mogelijk om twee (of meer) uitlees kastjes parallel op de P1 poort aan te sluiten. Deze post beschrijft hoe je dat toch voor elkaar kunt krijgen.
Continue Reading ..
Extending GPIO pins on your Micro Processor
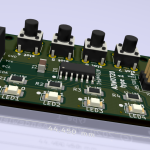
Somehow, no matter what micro processor I choose, I end up with too few GPIO pins for the projects needs or for what I want to do.
To end this run for GPIO pins for once and for all I decided I needed a cheep extension board that could be configured for Switches (input) and LED’s or other stuff (output). And while at it I thought some extra logic would be nice.
Continue Reading ..
ESP8266 ticker
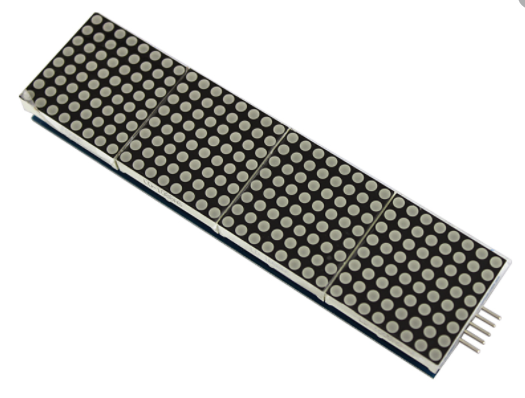
From the moment I saw the MAX7219 Dot matrix modules I wanted to build a news ticker. In addition to the messages to be entered by myself, I want the ticker to display the current weather and news items from the Internet and, of course, the time must also be shown!
Continue Reading
Controlling lots of relays with only two GPIO pins
I recently worked on a project that needed to control a lot of relays. There are these nice boards with 8 or 16 relays that are well suited for my purpose.
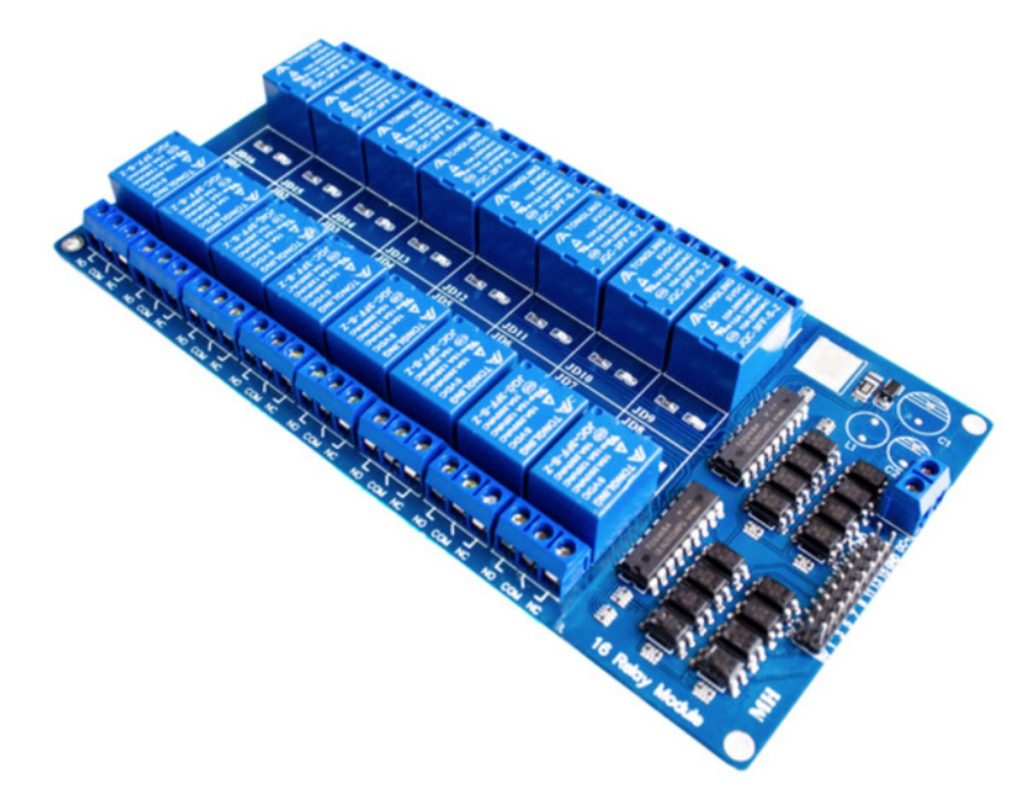
But you need a lot of wires and GPIO pins to control all those relays. So I came up with a piggyback board that uses I2C to control the relays.
You can now control as many of these boards as you like (well, there is a maximum address range of 127 I2C devices, but still 2000 relays with only two GPIO pins .. wauw!) Continue Reading
I2C Rotary Encoder
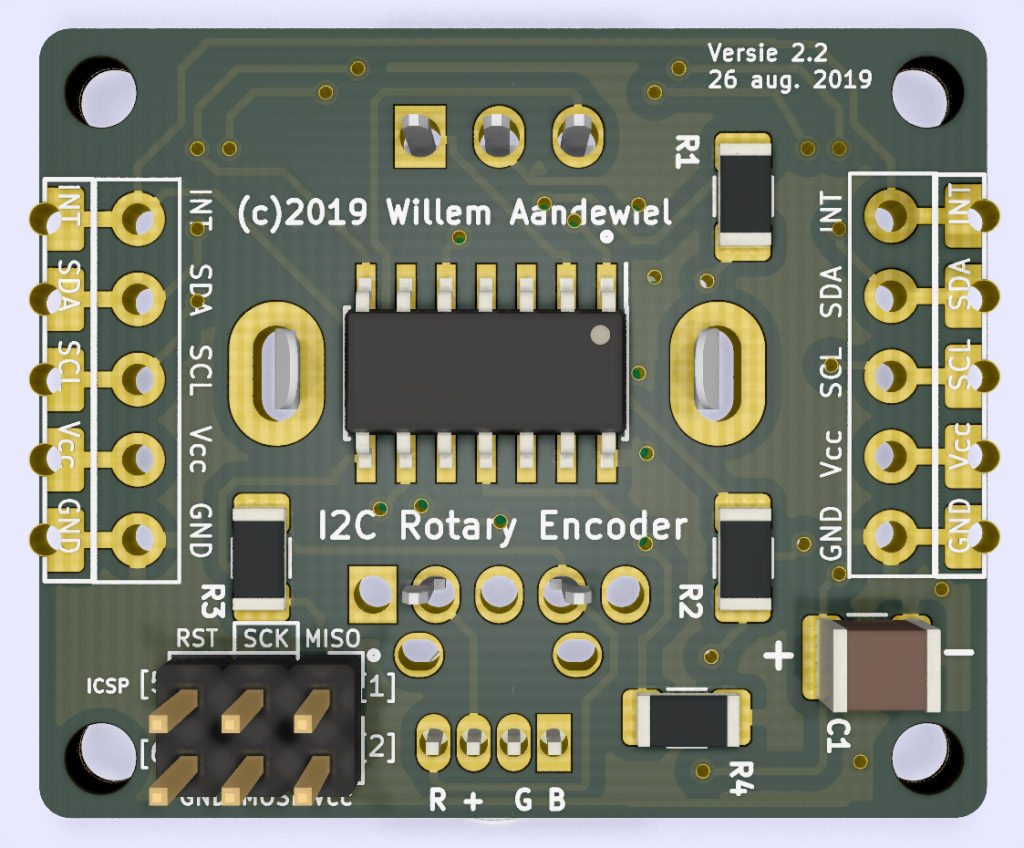
A Rotary Encoder is a device that looks (physically) like a potentiometer but it does not vary the resistance between two/three connectors. Instead it gives pulses when the axle is rotated. Another difference with the potentiometer is that you can rotate the axle over 360° (in fact, there is no limit in how many degrees you can rotate the axle). Continue Reading
Universal InfraRed Learning IoT Remote
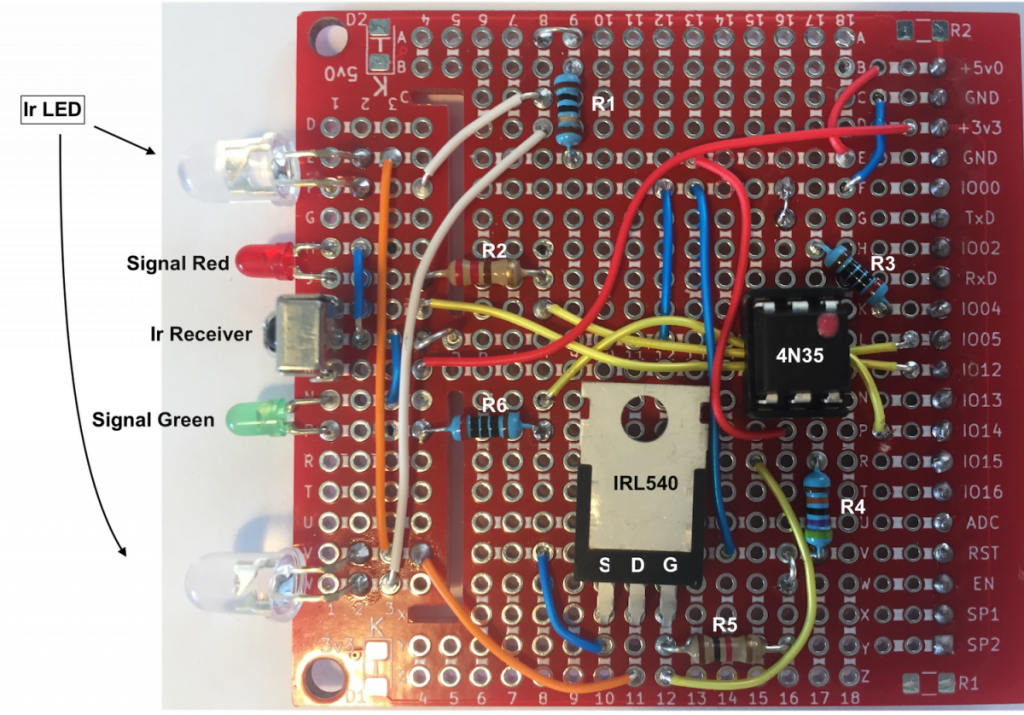
In this post I will guide you through the process of building a device that can capture Infrared-messages from an Infrared remote controller (learning mode), and resend the captured Infrared-messages (sending mode). Continue Reading.
Slimme Meter Uitlezer
Zo langzamerhand zijn alle huizen in Nederland uitgerust met een “Slimme Meter”. Hoog tijd dus om een project te starten om deze Slimme Meter thuis uit te kunnen lezen en de gegevens op een door jouw gewenst formaat te presenteren.
Dit project wordt niet meer actief ontwikkelt. De vervanger is de DSMR-logger32 met de ESP32 processor (zie de post “Crisis? What crisis? (nieuwe Slimme Meter reader)“.
Op 15 juni 2021 is v3.0.1 van de DSMRloggerAPI firmware gereleased!
Deze zgn. “One Fits All” release maakt het overbodig om voor verschillende soorten Slimme Meters (DSMR 2+, 3+, 4+ 5+ en de Belgische varianten hiervan) aangepaste firmware te gebruiken. Alle (mij bekende) Slimme Meters kunnen met deze éne release uitgelezen worden.
> DSMR-logger v4
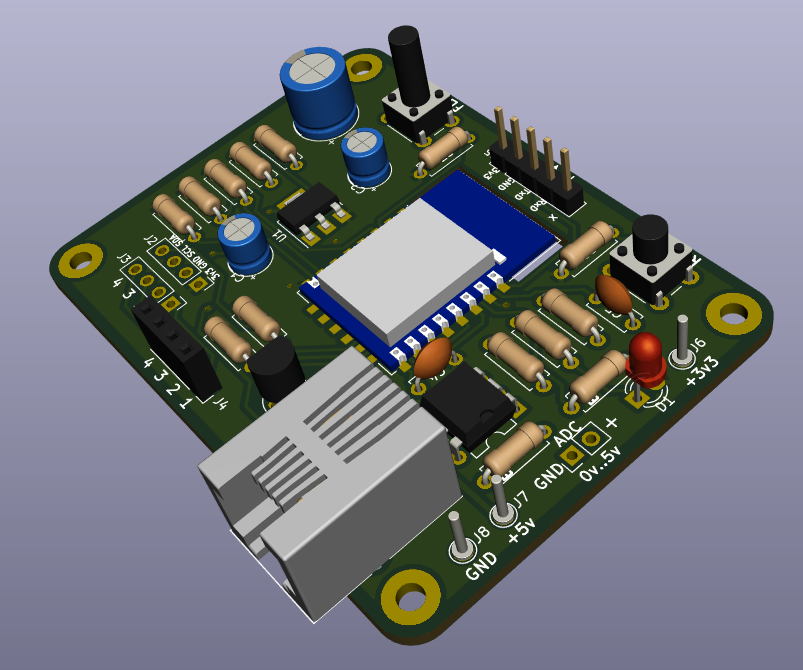
Aan de hand van de vele reacties van de mensen die het project hebben nagebouwd en mijn eigen ervaring met versie 3 van de DSMR-logger vond ik het tijd worden om een nieuwe versie van de hardware en de firmware te ontwikkelen.
Lees verder.
> Nieuwe firmware voor de DSMR-logger v4
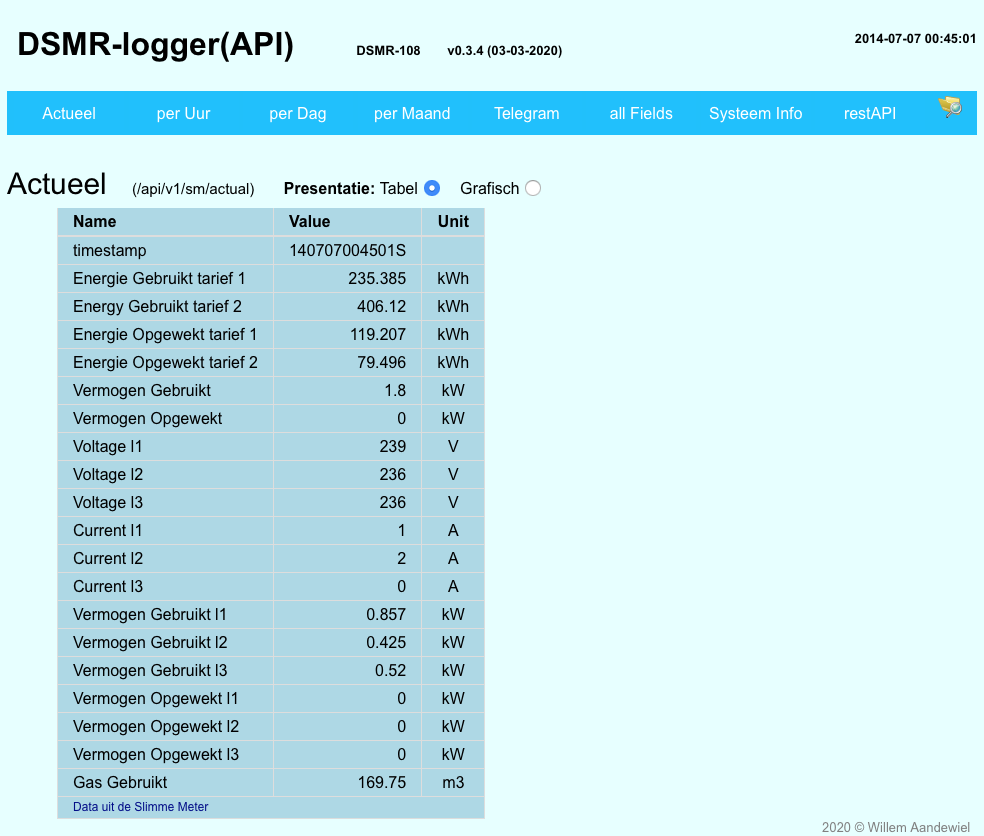
De nieuwe firmware voor de DSMR-logger is “helemaal into” restAPI‘s. Dit is een eenvoudig protocol waarmee gegevens uit de DSMR-logger kunnen worden opgevraagd.
De nieuwe firmware laat het hele idee dat het “alles zelf moet doen” los en laat ingewikkelde- en tijdrovende taken (bijvoorbeeld het opbouwen en tonen van een GUI) aan anderen over. Hierdoor is de firmware compacter en sneller geworden. Uiteraard zorgt de nieuwe firmware wel voor het opbouwen van historische bestanden maar doet dit nu in zgn. RING-bestanden die veel robuuster en sneller zijn dan de sequentiële (PRD) bestanden die in vorige versies van de firmware worden gebruikt. Wat is gebleven is de intergratie met MQTT.
Lees verder.
Aan de slag met de ESP8266
In deze post beschrijf ik hoe je de Arduino IDE voor de populaire ESP8266 processor-bordjes geschikt kunt maken. Hoewel al deze informatie gewoon van internet af te halen is blijkt het voor veel mensen, die de Arduino IDE of de ESP8266 bordjes net ontdekt hebben, overweldigend. Lees verder.
Solid State Tape Device for the (micro) KIM
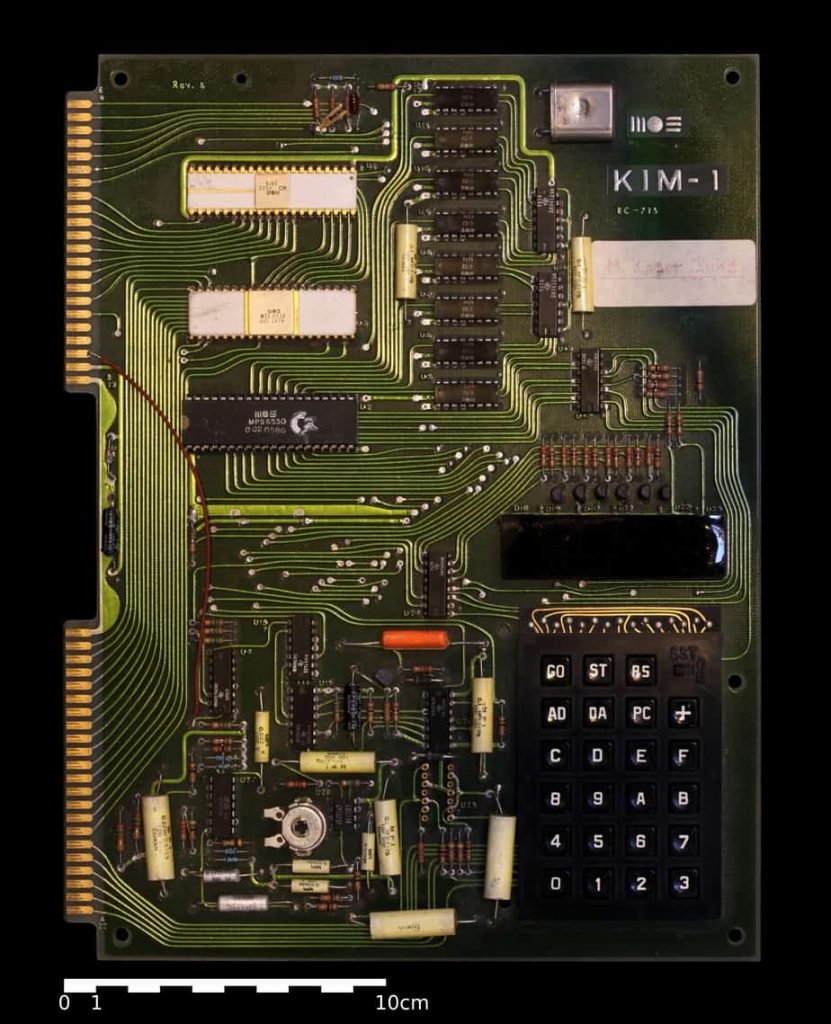
In 1976 I bought my first computer. That was at a time when there was no internet, there where no PC or Apple’s! Computers where meant for big company’s like insurance compagnies and multi nationals. No one had a computer at home. It was the time of the Homebrew Cmputer Club and Byte magazine. I had a Byte magazine subscription and at a certain point there was an advertisement in it from MOS-Technology for a single board computer called the KIM-1. Continue Reading.
NEW IMPROVED VERSION 2.0! Faster, Better, Nicer! Read all about it.
There is also a 32kb Memory Expansion Board!
BeeHave – a beehive data logger
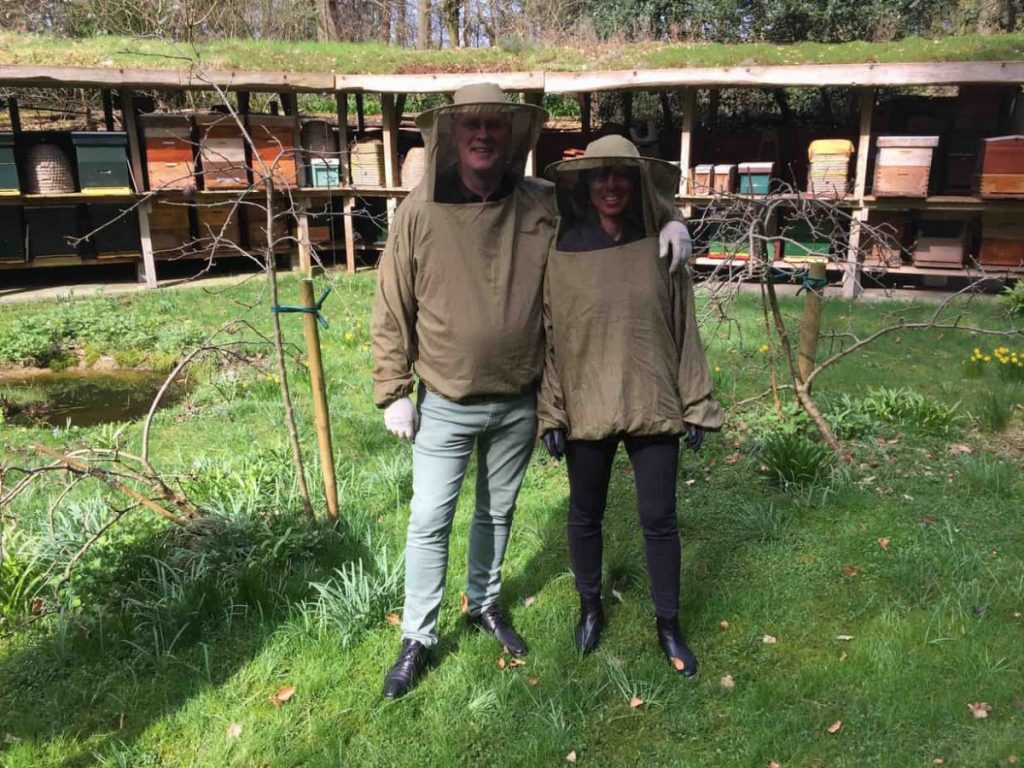
The objective of this project is to create a device that will measure the temperature inside the beehive at certain intervals, for instance every one, two, three, four or six hours. Continue Reading.
Eenvoudige Programmer voor de ESP-01 (ESP8266)
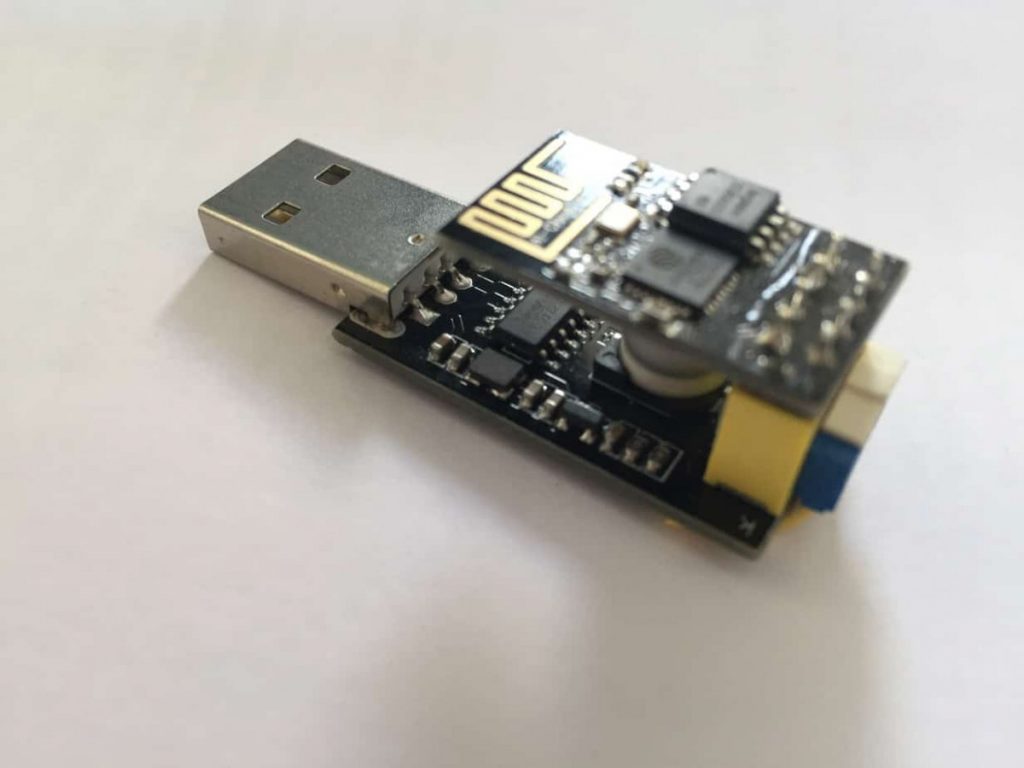
Om de ESP-01 te kunnen programmeren heb je een programmer nodig. Een andere, goedkope oplossing is, als je al een Arduino hebt, een speciaal programma in een Arduino-UNO te laden en dan met losse draadjes een aantal aansluitingen te maken tussen de Arduino en het ESP-01 bordje.
Het is makkelijker om een “ESP-01 to USB Adapter” te kopen en hier een kleine modificatie op aan te brengen. Lees verder.
Can you contact me to discuss your P1 devices. thanks
Hi Richard,
I send you a PM
Ok. You have my email? Thanks
Yes!
Beste Willem,
Ik kom graag in contact met je voor het ontwikkelingen van een P1 poort tester. Helaas zijn deze niet verkrijgbaar waardoor wij momenteel vast lopen.
Gezien jou blog kunnen wellicht iets duurzaams ontwikkelen tegen fair prijs voor installatiepartijen.
Mecit, ik stuur je een PM!
Geachte heer Aandewiel, Beste Willem
Ter info:
Ik heb de DSMR logger zonder problemen werkend op een XS210 ESMR5 slimme meter.
Zie de bijlage.
Met vriendelijke groet,
Manuel van Amerongen
Dag Willem,
Een vriend wees me op deze site omdat ik uitleesunits maak voor de groepsmeter van GPX. Misschien is het aardig wat uit te wisselen. We werken uitsluitend open source, en GPX is non-profit. Demo groepsmeter.
Zie ook Github
Ben benieuwd.
Mvg, Egbert Bouwhuis, Arnhem
Hallo Egbert,
Wat een mooi project!
Ik stuur je een PM
Dag Willem,
Ik kom bij toeval op deze site terecht en heb een probleempje.
Al mijn afstandsbedieningen zijn geprogrammeerd in een universele van het type Logitech. Deze A.B. kan ook opdrachten leren.
Van álle apparaten lukt dit maar niet van de Horizon Next Box van Ziggo.
Het blijkt, dat dit apparaat aangestuurd wordt met een ander signaal dan InfraRood maar wél draadloos.
Ik heb ook de mogelijkheid om een RAW opdracht te leren maar wat is dat ?
Heb je enig idee hoe dit moet worden opgelost?
Bij voorbaat dank.
Frans Habets
Volgens mij werkt de Ziggo box met bluetooth en/of WiFi.
hallo misschien een vreemde vraag voor u ik doe in ijsmachines de aandrijving geschied door een elektromotor om het ijs van een cilinder af te schrapen de koude hiervoor word geleverd door een compressor .deze word in en uitgeschakeld door een micro schakelaar op de aandrijfriem middels een trekveren stelsel van elektromotor .werkt op trekkracht deze zijn complex en moeilijk in te stellen en nooit constant mijn vraag is het mogelijk om met een sensor op de as van de motor het toeren tal te meten en deze bij een verlaagt toerental te laten schakelen bv de motor maat onbelast 1200 rpm bij een trekkacht met bv 900 rpm moed hij schakelen zodat de koelmotor uitschakeld en weer een oplopend toerental bv 900 rpm weer inschakeld dez diff moed instelbaar zijn de motoren zijn 1,5 pk en worden met 380volt geschakeld dmv vermogens relais met een spoelspanning van 220 volt als u verder info wilt kan ik wat info toesturen dat het wat duidelijker word of telefoneren bvd gerard vermeulen
Gerard,
Wat jij wil is zeker mogelijk.
Ik stuur je een PM met wat vragen voor aanvullende informatie (foto’s, schema’s enz.)
Beste Willem,
Ik kom u net tegen op internet. Misschien kunt u mij helpen met het volgende;
Ik heb een floww kokend water kraan. Daar zit wsl. een rotary encoder in met een RGB led. Er zit een USB kabel (grote usb) aan de kraan. Deze werkt met een HUB (400,-), die ik niet heb en te prijzig vindt.
Weet u hoe ik de kraan werkend kan krijgen met eenvoudige electronica?
Het is voldoende als ik een paar kleppen kan aansturen voor kokend water. Kunt u wellicht e.e.a. samenstellen en tegen welke kosten?
Ik hoor graag van u.
Met vriendelijke groet,
Marco
Toffe projecten Willem !Je bent een topper!
Keep on the good work.
Misschien is het ook eens interessant om een smart smoke detector projectje op te zetten met een esp8266 ? Dit zou ik in elk geval ook heel erg interessant vinden.
Mvg Tino
Hi Tino,
Bedankt voor het compliment.
Over je voorstel ga ik zéker nadenken.